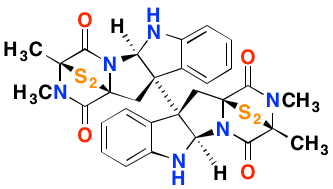
Organic Chemistry
Organic chemistry is the chemistry of carbon compounds. Due to the ability of carbon to form chains of atoms, and for other atoms or groups of atoms to be attached to these chains, there are a huge number of carbon compounds. All organic compounds contain carbon together with one or more other elements such as hydrogen, oxygen, nitrogen, sulphur and the halogens.
Normally every compound of carbon is an organic compound. Even after discovering that these compounds could be synthesized in the laboratory, the definition that they are organic (of organic nature, that is, they originate from living things) has been retained. However, for conventional and historic reasons, some of the simpler compounds such as carbon dioxide (CO2) carbonates, carbon monoxide (CO3) are usually studied with other non-carbon compounds in Inorganic Chemistry.
Difference between Organic from Inorganic Chemistry
Distinguish organic from inorganic chemistry
Organic chemistry is the chemistry of carbon compounds. All organic compounds contain carbon and other elements such as H, O, N, S and the halogens. Normally every compound of carbon is an organic compound. Examples of organic compounds/substances are plastics, milk, carbohydrates, lipids, proteins, sugar and hydrocarbons. Inorganic substances includes table salt, CO2, diamond, iron and water.
The Importance of Organic Chemistry in Life
Explain the importance of organic chemistry in life
Carbon is the most unusual atom. It has the ability to join up to itself and form very long chains of atoms. Without this ability, life on Earth would not exist because the molecules that make our bodies contain mostly long chains of carbon atoms.
All living things contain carbon compounds. Raw materials such as oil and coal, derived from living things, are also based on carbon. Our modern society is very much dependent on organic chemistry to make the fuels and materials that we use in every day of our lives. In particular, polymers, large molecules obtained from alkanes, have very widespread use. Without alkanes from crude oil our transport system would be impossible.
We also need various fractions obtained from crude oil (petrol, diesel, kerosene, oil, natural gas, etc.) to run motor vehicles and other machines to simplify our work and life.
In brief, the compounds obtained from crude oil have thousands of different uses, for example:
- some are used as fuel or converted into fuels;
- some are used for making detergents, dyes, drugs, paints, and cosmetics; and yet some are used for making polyethene, polyvinyl chloride (PVC) and other plastics.
The Origin of Organic Compounds
Explain the origin of organic compounds
Fossil fuels were formed in the Earth’s crust from material that was once living. Coal comes from fossils of plant material. Crude oil and natural gas are formed from bodies of marine microorganisms. The formation of these fuels took place over geological periods of time (many millions of years).
Crude oil is one of the world’s major natural resources. The oil is the result of a process that began up to 400 million years ago. Prehistoric marine organisms died and sunk to the sea bed and were covered by mud and sand. The change into crude oil and natural gas was brought about by high pressure, high temperature and bacteria acting over millions of years. The original organic material broke down into hydrocarbons. Compression of the mud above the hydrocarbon mixture transformed it into shale. Then geological movements and pressure changed this shale into harder rocks, squeezing out the oil and gas. The oil and gas moved upwards through the porous rocks, moving from high- pressure to low-pressure conditions. Sometimes they reached the surface, but often they became trapped by a layer of non porous rock. Reservoirs of oil and gas were created. These reservoirs are not lakes of oil or pockets of gas. Instead, the oil or gas is spreadthroughout the pores in coarse rocks such as sandstone or limestone the same as water is held in a sponge.
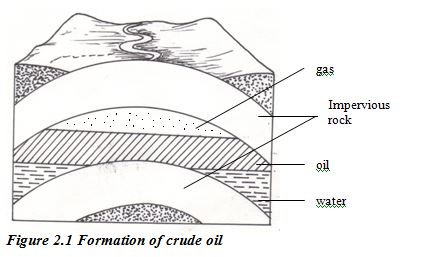
The Fractional Distillation of Crude Oil
Describe the fractional distillation of crude oil
Crude oil from an oilfield is separated from impurities such as sand and water and is pumped through pipelines to the refinery. At the refinery, fractional distillation is used to separate the crude oil into several fractions, each fraction containing several hydrocarbons which boil within a certain range of temperatures. These different boiling points are roughly related to the number of carbon atoms in the hydrocarbon (Table 2.1)
Separation of the hydrocarbons takes place in a fractionating column (fractionating tower). At the start of the refinery process, crude oil is preheated to a temperature of 350–400°C and pumped in at the base of the tower. As it boils, the vapour passes up the tower. It passes through a series of bubble caps and cools as it rises further up the column. The different fractions cool and condense at different temperatures, and therefore at different heights in the column. The fractions condensing at the different levels are collected on trays.
Thus, vapour is rising and liquid falling at each level in the tower. As a result very efficient fractionation occurs. Liquid is taken off at several different levels, the higher the level, the lower the boiling point of the fraction removed. Figure 2.2 shows the process of separation of crude oil into different fractions.
After fractional distillation, impurities are removed. The commonest impurity is sulphur, which is removed and used to manufacture sulphuric acid. If petrol (gasoline) containing sulphur is not purified before it is used in an internal combustion engine, the exhaust fumes will contain oxides of sulphur (SO2 and SO3). These are poisonous gases and will pollute the environment.
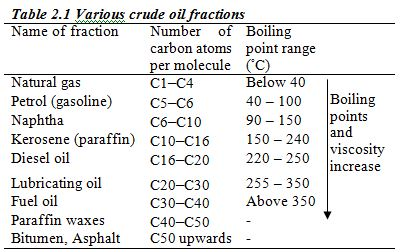
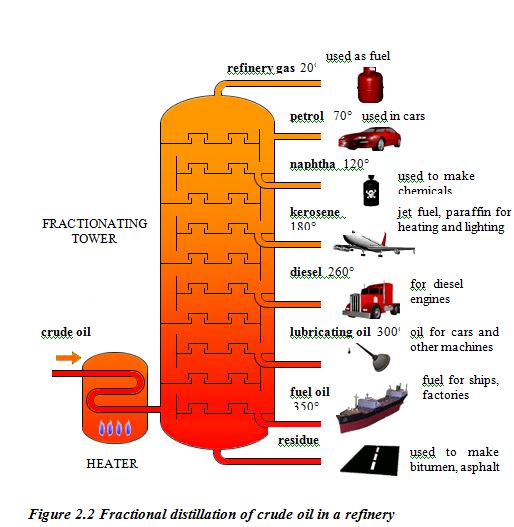
Uses of different petroleum fractions
1. Natural gas (refinery gas). The gas fractions consist of mainly methane, ethane, propane, and butane. The methane and ethane are usually burnt as fuel. The propane and butane are liquefied and are distributed in high pressure gas cylinders and tanks to be used for lighting and heating purposes.
2. Petrol (motor gasoline). It is mainly used as a fuel in internal combustion engines in motor vehicles. It is also used as a solvent for grease stains and paints.
3. Naphtha. It is used as a raw material for making many chemicals and plastics.
4. Kerosene (paraffin). It is used in homes as a fuel in paraffin lamps and stoves for heating, lighting and cooking food. However, in addition to its domestic use, it is used as a fuel for jet engines in aeroplanes. It is also used as a detergent.
5. Diesel oil. It is used as a fuel in diesel engines (e.g. in diesel train engines, tractors, lorries, diesel car engines).
6. Lubricating oil. It is used to make petroleum jelly (e.g. Vaseline). It is also used as oil for lubricating moving parts of cars and other machines.
7. Fuel oil. It is used as a fuel for power stations, ships and factories.
8. Paraffin waxes. They are used to make candles, polishes and waxed papers. They are also used in water proofing and as grease.
9. Asphalt, bitumen. They are used to make protective coatings for road surfaces and concrete roof tops, and also as binding agents for roofing sheets.
Hydrocarbons
Hydrocarbons are compounds containing hydrogen and carbon and no other element. That is, a hydrocarbon has the molecular formula CxHy, x and being whole numbers. For example, methane (CH4) ethane (C2H2) and benzene (C6H6) are hydrocarbons.
The three Families of Hydrocarbons
Classify the three families of hydrocarbons
In order to simplify the study of these compounds, chemists have grouped them into families. The members of each family have characteristic chemical properties and graded physical properties, such as boiling point, etc. The main family members of hydrocarbons that we shall study at this level are the alkanes, the alkenes and the alkynes.
Alkanes
The members of this group of hydrocarbons are distinguished by possessing the general molecular formula Cn H2n+2, where is 1, 2, 3, etc., for successive members of the group. The first member of the series (n = 1) is methane (CH4) and the second (n = 2) is ethane (C2H6). Both are gases at room temperature and pressure. This general formula can be used to work out the formula of any other alkane if we know the number of carbon atoms it consists of. The following table gives the molecular formula and name of the first few alkanes, plus an indication of some of their physical properties.
Table 2.2 Formulae and physical properties of some alkanes
name | Molecular formula | Melting point (oC) | Boiling point (oC) | Density (gcm–3) |
Methane | CH4 | –183 | –162 | gas |
Ethane | C2H6 | –172 | –89 | gas |
Propane | C3H8 | –188 | –42 | gas |
Butane | C4H10 | –135 | –1 | gas |
Pentane | C5H12 | –130 | 36 | 0.626 |
Hexane | C6H14 | –95 | 69 | 0.659 |
Heptane | C7H16 | –91 | 98 | 0.684 |
Octane | C8H18 | –57 | 126 | 0.703 |
Nonane | C9H20 | –54 | 151 | 0.718 |
Decane | C10H22 | –30 | 174 | 0.730 |
Structure of alkanes
In the alkanes, all carbon atoms show a covalency of four. Table 2.3 shows the structures of the first five members of the alkanes.
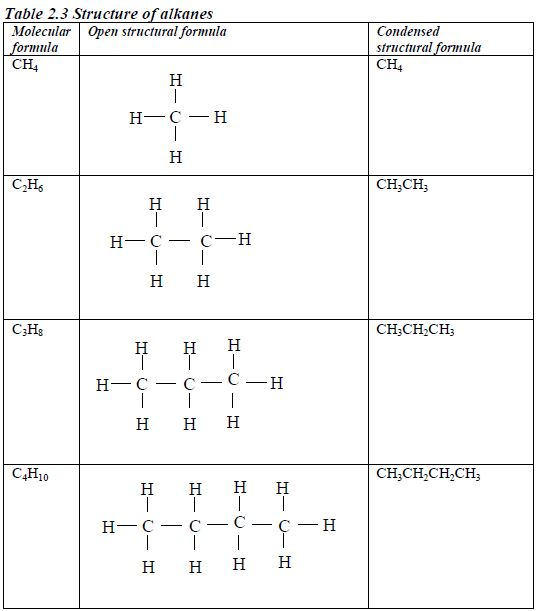
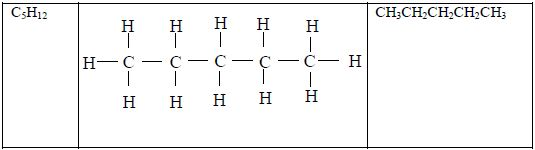
The Homologous Series of the Three Families of Hydrocarbons
Write the homologous series of the three families of hydrocarbons
A homologous series is a series of compounds with similar chemical properties which differ by –CH2. Such series has the following characteristics:
- All members obey the general molecular formula, e.g. for alkanes Cn H2n+2, alkenes Cn H2n and alkynes, Cn H2n-2.
- Each member differs, in molecular formula, from the next by CH2, e.g. alkanes are CH4, C2H6, C3H8, and so on.
- All members show similar chemical properties.
- The general properties of members change gradually in the same direction along the series, e.g. in alkanes, boiling points and freezing points rise with increase in the number of carbon atoms (CH4 – a gas; C5H12 – a liquid; C20H42 – a solid at ordinary temperatures and pressure).
- General methods of preparation are known which can be applied to any member of the series. Other homologous series are alcohols, CnH2n+1 and carboxylic acids, CnH2n+1COOH.
Nomenclature of alkanes
The term nomenclature means naming. Organic compounds are named using IUPAC system of nomenclature. IUPAC stands for International Union of Pure and Applied Chemistry.
Rules of Nomenclature
1. Name the longest unbranched carbon chain. For example, consider the following compound:
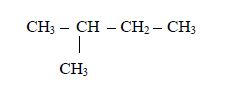
In this compound, the longest unbranched chain is
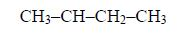
The longest chain is, therefore, butane.
2. Name the functional groups. Functional groups are branches of the main carbon chain. These are sometimes called alkyl groups.
Examples of alkyl groups are as follows:
- CH3 – methyl
- CH3CH2 – ethyl
- CH3CH2CH2 – propyl
- Cl – chloro
- Br – bromo
- I – iodo
- F – flouro
3. Give the position(s) of the functional group(s) using lower numbers when possible. Consider the following example:
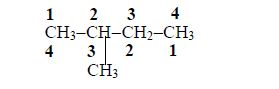
There are two possibilities of naming the above compound but only one is correct.
- 2 – methylbutane
- 3 – methylbutane
The first name is correct because it bears the lowest number (2). The second name is wrong because it bears a large number (3).
4. Names of the functional groups are named in alphabetical order in the final name.
Example
Consider the following compound:
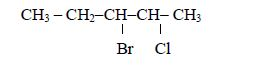
The name of the compound is: 3–bromo–2–choloropentane
Note: We start with bromo and not chloro because b comes before c in alphabet (a, b, c, d …)
5. If there are identical functional groups, use the prefixes di (2), tri (3), tetra (4), penta (5), etc. These prefixes do not account for alphabetical order.
Examples:
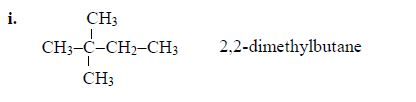
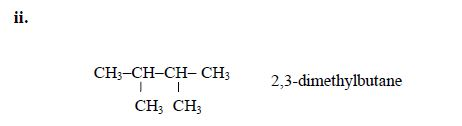
6. Commas separate numbers and hyphens separate numbers from words.
The Concept of Isomerism
Explain the concept of isomerism
Isomers are organic compounds with the same molecular formula but different structural formulae.
Isomerism is the occurrence of two or more compounds with the same molecular formula but different molecular structures (structural formulae).
Isomers of the same molecular formula have different physical and chemical properties because of structural differences.
Structural Formulae of all Isomers of Hydrocarbons up to Five Carbon Atoms
Write structural formulae of all isomers of hydrocarbons up to five carbon atoms
Example 1
1. Butane The two isomers of butane, with molecular formula C4H10, are as shown below. Isomers can be presented either in an open formula (structure) as follows.
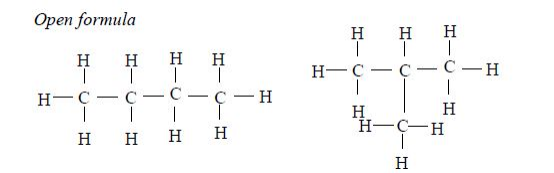

Example 2
Pentane
The three isomers of pentane showa open formula asfollows:
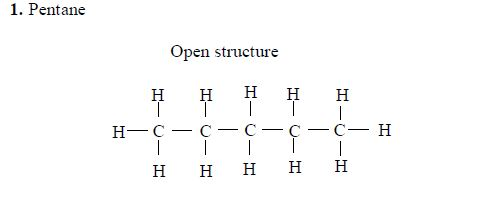
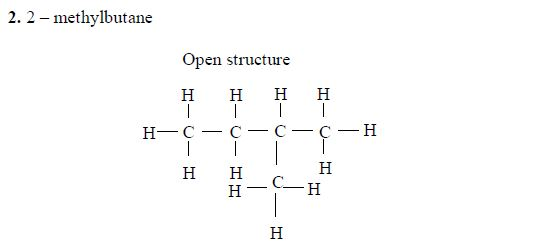
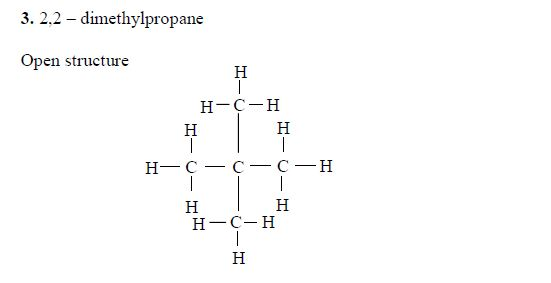
Isomers of Hydrocarbons up to Five Carbon Atoms
Name the isomers of hydrocarbons up to five carbon atoms
Example 3
alkanes showing isomerism
1. Butane The two isomers of butane, with molecular formula C4H10, are as shown below. Isomers can be presented either in an open or condensed formula (structure).
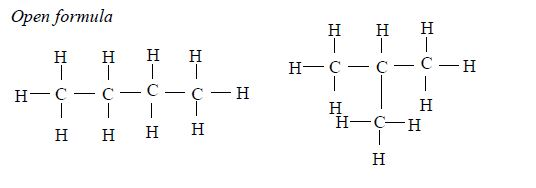
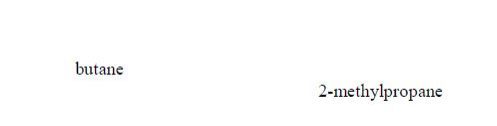
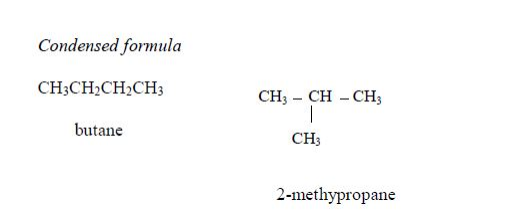
Note: Remember that CH4, C2H6 and C3H8 have no isomers because they cannot form alkyl groups, that is, they cannot be branched. Isomerism in alkanes starts from an alkane with four carbon atoms (butane) in its structure (C4H10).
2. Pentane
The three isomers of pentane are shown as follows:
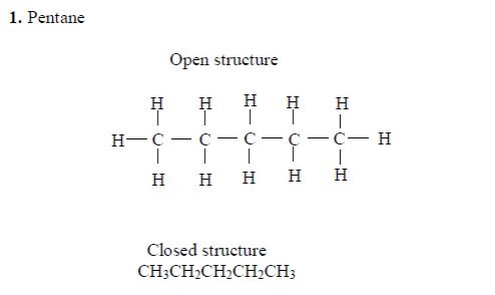
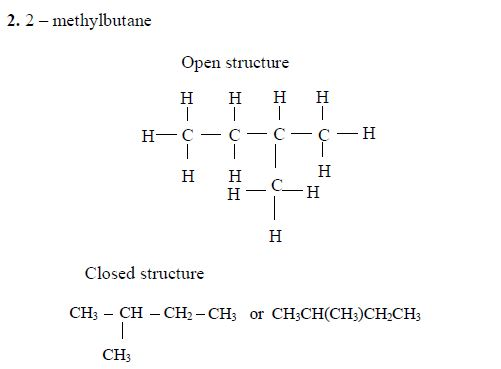
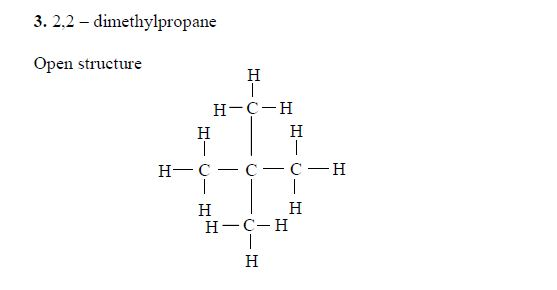
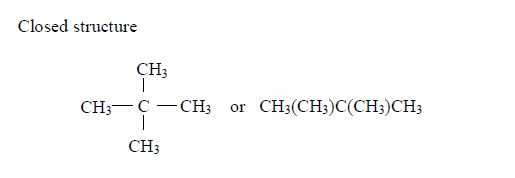
Alkenes
Alkanes are members of a homologous series of hydrocarbons with general molecular formula Cn H2n where n = 2, 3, 4, etc. The common structural feature is the presence of a carbon–carbon double bond:
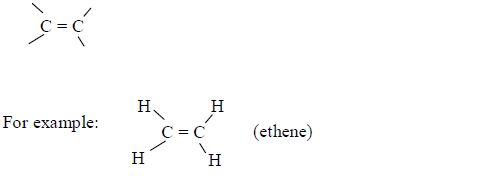
There is no member of this series for n = 1 since there needs to be at least two carbon atoms present to have a double bond. Table 2.3 shows the first five members of alkenes.
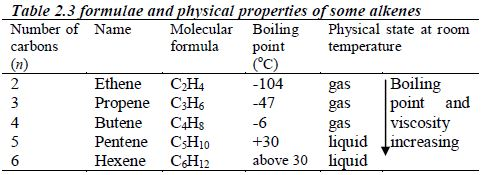
Structure of alkenes
Alkanes are characterized by possessing one or more carbon to carbon double bond(s) (C=C) in the carbon chain. Alkenes are unsaturated hydrocarbons because it is possible to break the double bond and add extra atoms to the parent molecule.
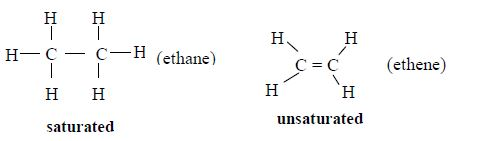
Table 2.4 shows the structures of the first five members of the homologous series of alkenes.
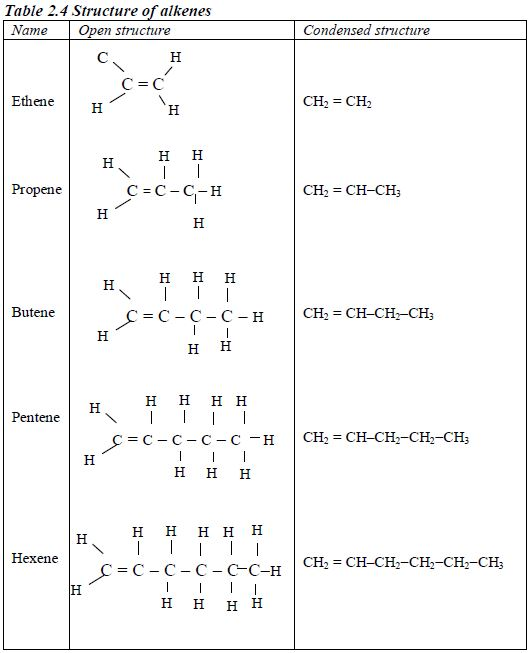
Nomenclature of alkenes
Rules for naming alkenes
- Names of alkenes have the ending –ene.
- The double bond is given the number of the carbon atom where it begins.
- In addition to the rules discussed earlier, the position of the double bond must be included in the name .Examples: CH3–CH=CH3 prop-1-ene , CH3–CH=CH–CH3 but-2-ene
- Double bonds are given the lowest number possible, usually lower than the functional groups.
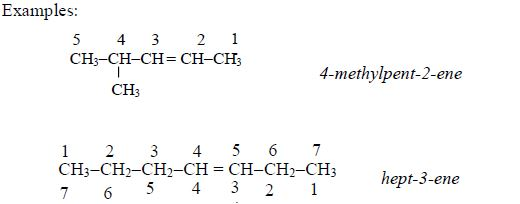
Counting from the left to right, the double bond (=) is on carbon number 4 and from the right to left, it is on carbon number 3. Because the double bonds should be given the lowest umber possible, the name of the compound is, therefore, hept-3-ene instead of hept-4-ene.
Isomerism
Alkenes, like alkanes show branching isomerism, for exampleCH3–CH=CH–CH3 (pent-1-ene) can be branched into (3-methylbut-1-ene) as follows:

They also show positional isomerism due to different positions of the double bond in the molecule, for example, pent-1-ene and pent-2-ene are both the isomers of pentene (C5H10):
CH2=CH–CH2–CH2–CH3 pent-1-ene
CH3–CH=CH–CH2–CH3 pent-2-ene
Other examples include:
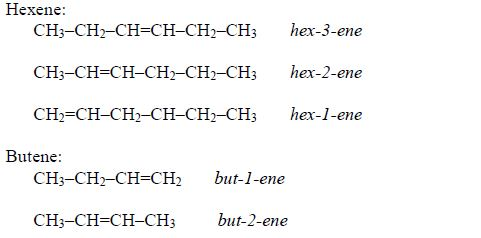
Alkynes
These are homologous series of hydrocarbons with the general formula CnH2n-2. The common structural feature is the presence of a carbon-carbon triple bond (CC). There is no member for n = 1 because, as for the alkenes, there needs to be at least two carbonatoms present to have a triple bond. Table 2.5 shows the first five members of the alkynes.
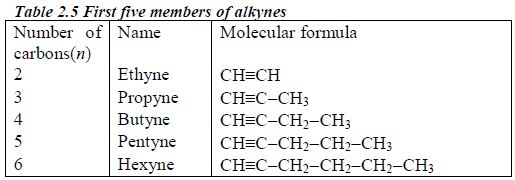
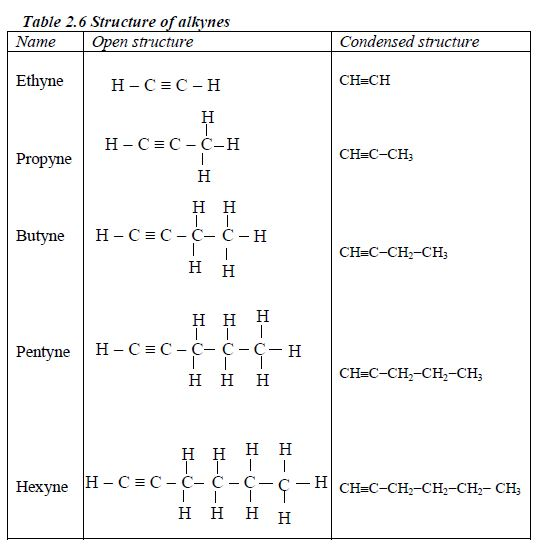
Nomenclature of alkynes
Rules for naming alkynes
- Alkynes have the ending –yne
- Triple bond is given the lowest number possible, usually lower than the functional groups.
- As for the alkenes the position of the triple bond must be included in the name.
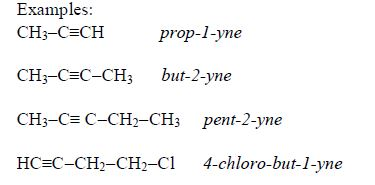
Isomerism
Alkynes show branching isomerism and positional isomerism. The alkyne with the molecular formula, C5H8, shows chain branching isomerism, for instance,
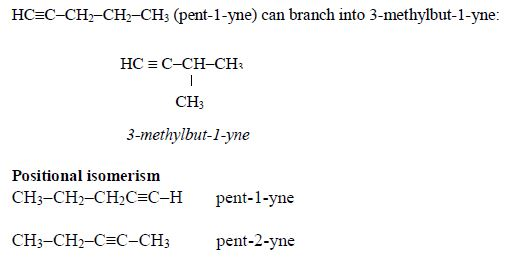
Properties of Hydrocarbons
The Physical Properties of Lower Hydrocarbons; Alkanes, Alkenes and Alkynes
Explain the physical properties of lower hydrocarbons; alkanes, alkenes and alkynes
Alkanes
- The first four alkanes are gases at room temperature, the next twelve (C5–C17) are liquids, and the rest are solids.
- The boiling and melting points of unbranched alkanes increase as the molar masses increase. The larger the molecule, the higher the boiling point or melting point.
- Branched alkanes have lower boiling points than the unbranched alkanes,
- Alkanes are very sparingly soluble in water but they easily dissolve in organic solvents.
- In an alkane molecule, each carbon atom forms four single covalent bonds. This means that alkanes are saturated hydrocarbons.
Alkenes
Here again we find that alkenes posses physical properties that arethe same as those of alkanes, i.e.
- They are insoluble in water but soluble in organic solvents suchas benzene, ether and chloroform.
- They are less dense than water.
- The boiling point increases with increase in molecular weight i.e., increase in the number of carbon atoms.
Alkynes
The physical properties of the alkynes are essentially the same as those of the alkanes and alkenes.
The Concept of Saturated and Unsaturated Hydrocarbons
Explain the concept of saturated and unsaturated hydrocarbons
Hydrocarbons are classified into two distinct categories: saturated and unsaturated. Saturated hydrocarbons contain only one carbon-carbon single bond. Unsaturated hydrocarbons contain at least one carbon-carbon double or triple bond. The unsaturated hydrocarbons are more reactive and they contain fewer hydrogen atoms bonded to the carbon atoms than saturated hydrocarbons.
The Chemical Properties of Alkanes, Alkenes and Alkynes
Compare the chemical properties of alkanes, alkenes and alkynes
Alkanes
Alkanes are not as reactive as other hydrocarbons. However, they exhibit the following chemical properties:
Combustion: alkanes burn in a sufficient amount of air to form carbon dioxide and steam.

Cracking: this is a process in which large alkanes are broken down into smaller hydrocarbons.

Halogenation: under special conditions (sunlight or 300°C), halogens react with alkanes in a substitution reaction. A substitution reaction is a reaction in which one atom or group of atoms replaces another.
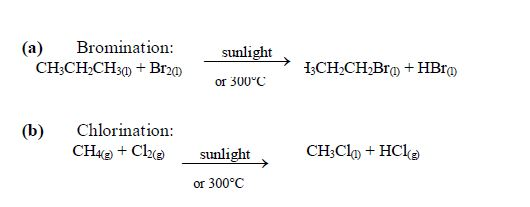
Alkenes
Alkenes are much more reactive than alkanes. This is because thedouble bond can break to form single bonds and add on otheratoms. Because the double bond allows them to add on moreatoms, alkenes are said to be unsaturated. The alkanes don‟t havedouble bonds and can‟t add on more atoms, so they are saturated.
Combustion: alkenes burn in sufficient supply of air (explodes)to form carbon dioxide and steam.

Addition reactions: Alkenes give a number of addition reactions in which two hydrogen atoms (or their equivalent) are taken intocombination per molecule to form a single product. The following are some of the addition reactions shown by alkenes:
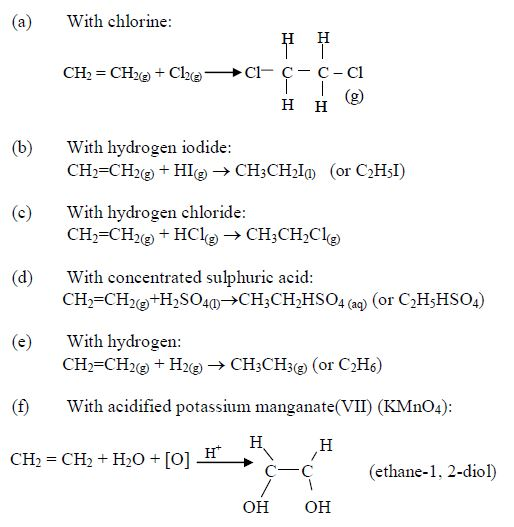
Alkynes
Addition: having carbon–carbon trip bonds, alkynes are unsaturated compounds. They give a number of addition reactions:
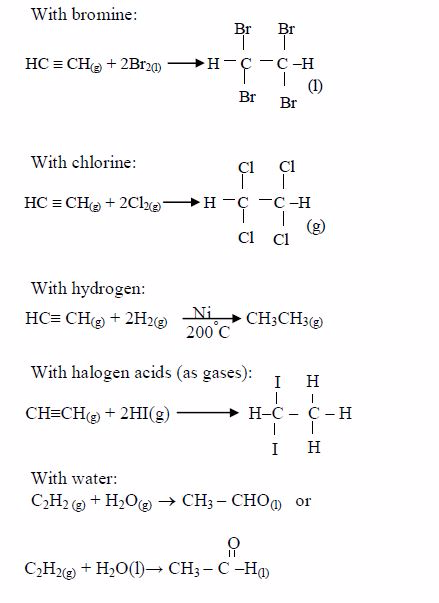
With acidified potassium manganate (VII) solution: At room temperature, with shaking, ethyne quickly decolourizes this solution (i.e. reduces it) with formation of ethanedioc acid
Polymerization

Combustion:

Salt formation:

This is a test for terminal alkynes, i.e. those with C≡C at the end. For those with C≡C at the centre, no reactions take place e.g. CH3–CH2–C≡C—CH2–CH3 + AgNO3→ No reaction!
Alcohols
Alcohols form a homologous series of general molecular formula, CnH2n+2, where n = 1, 2, 3, etc. for successive members of the group. The hydroxyl group, OH, is the characteristic functional group of the alcohols.
Preparation of Ethanol in the Laboratory
Prepare ethanol in the laboratory
Laboratory preparation of an alcohol involves heating a haloalkane with an aqueous alkali. Ethanol is prepared by heating a mixture of bromoethane and sodium hydroxide solution. The reaction proceeds as follows:

The ethanol, as indicated in the equation will be in solution. It canbe isolated by distillation.
Ethanol can also be prepared by fermentation of glucose wherebyyeast produces an enzyme called zymase which acts as an organiccatalyst to catalyse the degradation of glucose.
For substantial fermentation to take place, a mixture of glucose and yeast is left in a warm place for a considerable length of time.
The equation for the reaction taking place is:
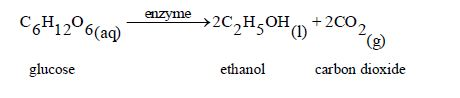
The Homology of Alcohols up to Five Carbon Atoms
Write the homology of alcohols up to five carbon atoms
Alcohols are named as if they are derived from alkanes by the replacement of hydrogen atom by the hydroxyl group (–OH). The lowest two members containing one and two carbon atoms in the molecule, are, respectively, methanol and ethanol. Their structural formulae are shown below:
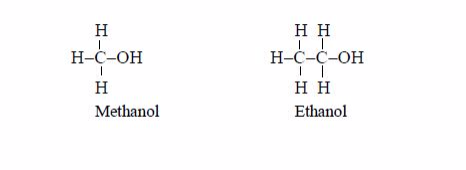
Other members of the series are as shown below:
Number of carbons per molecule (n) | Name | Formula |
1 | Methanol | CH3OH |
2 | Ethanol | C2H5OH |
3 | Propanol | C3H7OH |
4 | Butanol | C4H9OH |
5 | Pentanol | C5H11OH |
6 | Hexanol | C6H13OH |
7 | Heptanol | C7H15OH |
8 | Octanol | C8H17OH |
9 | Nonanol | C9H19OH |
10 | Decanol | C10H21OH |
The successive members of the series have molecular formulae which differ by CH2.
Like other series, alcohols exhibit isomerism. For instance, propanol (C3H7OH) can have two different structural formulae as exemplified below:
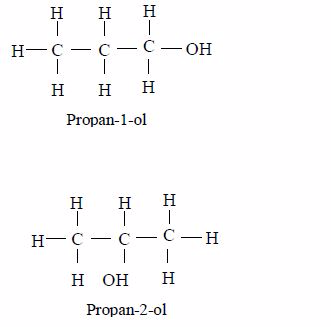
The first is called propan-1-ol because the OH group is on the first carbon atom.
The second is called propan-2-ol because the OH group is on the second carbon atom.
Structure of all Isomers of Saturated Alcohols up to Five Carbon Atoms
Write structure of all isomers of saturated alcohols up to five carbon atoms
Nomenclature of branched alcohols
Rules:
- For branched alcohols, the–OH group is given the lowest number than the alkyl group.
- The alcohol group (–OH) or the alkyl groups can be attached to different positions of the carbon chain. It is the different positions of these groups that result to the different names of the alcohol.
- The carbon chain may have several branches of different alkyl groups.
Examples:
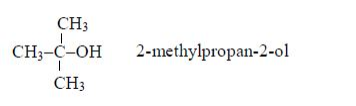
Note: Both the methyl (–CH3) and hydroxyl (–OH) groups are on carbon number 2. The longest unbranched carbon chain is
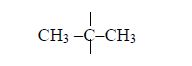
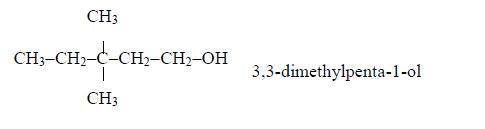
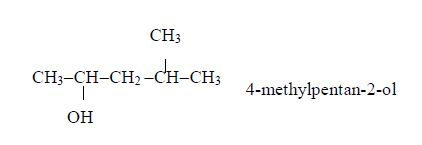
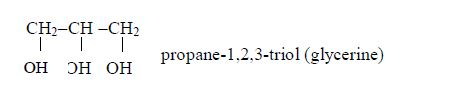
Isomers of Alcohols up to Five Carbon Atoms
Name all isomers of alcohols up to five carbon atoms
Alcohols exhibit chain branching isomerism as with all the other homologous series. In addition, they show an isomerism based on the position of the hydroxyl group (–OH) in the molecule.
Alcohols that have the formula CH3OH and CH3CH2OH have only one possible structure. They do not have isomers. The –OH group in ethanol, CH3CH2OH, is always on the last carbon atom.
With the formula CH3CH2CH2OH, however, the –OH group can either be attached to the first or second carbon of the carbon chain to give two isomers namely, CH3CH2CH2OH (propan-1-ol) and

Isomers of other members in the series are as follows:
Butanol: C4H9OH or CH3CH2CH2CH2OH
Isomers:
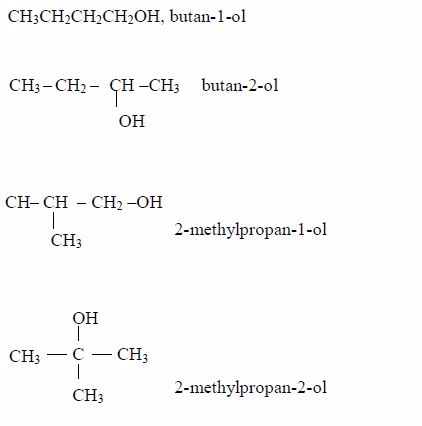
Pentanol: C5H11OH or CH3CH2CH2CH2CH2OH
ItsIsomers:
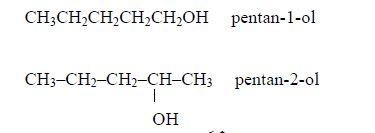
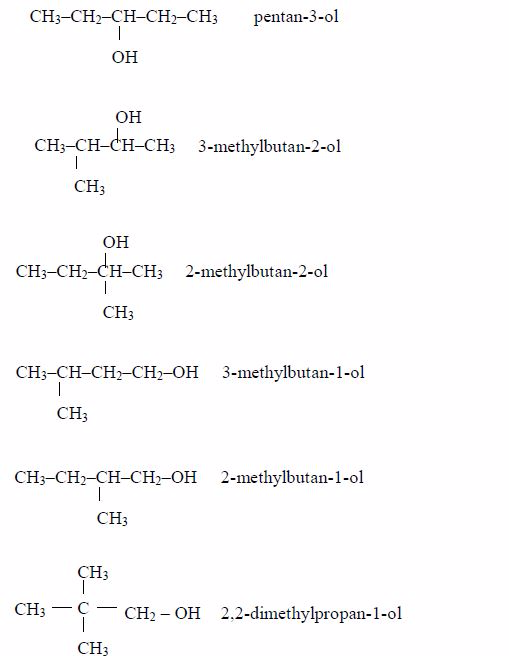
The Properties of Alcohol
Describe the properties of alcohol
Ethanol is the best known and most important of all alcohols. It has several different uses. Due to its diverse use, its name is used interchangeably with that of “alcohol” as if it represents all other classes of alcohols.
Physical properties of ethanol (alcohol)
- It is a clear, colourless liquid with a boiling point of 78°C.
- It is readily soluble in water and it mixes completely with it (miscible).
- It evaporates very easily when exposed to air (it is a very volatile liquid).
Chemical properties of ethanol
Reaction with oxygen (combustion)
It burns well in oxygen giving carbon dioxide and water, and plenty of heat.

This reaction is the basis of its use as a fuel.
Reaction with sodium metal
Ethanol reacts vigorously with sodium to produce sodium ethoxide and hydrogen. However, the reaction is not as vigorous as the reaction between sodium and water.

Methanol reacts similarly to produce sodium methoxide and hydrogen:

Reaction with ethanoic acid (esterification)
Alcohols react with organic acids to form sweet-smelling oily liquids known as esters.

The symbol, H+, indicates that the reaction takes place in acidified conditions. Normally dilute sulphuric acid is added as a catalyst for this esterification reaction.
Reaction with concentrated sulphuric acid
Concentrated sulphuric acid dehydrates ethanol to give ethene. The reaction takes place at 180°C.

Reaction with phosphorus pentachloride
Ethanol reacts vigorously with phosphorus pentachloride to give chloroethane, phosphorus oxychloride and hydrogen chloride.

Hydrogen chloride is evolved as white fumes, and this reaction is used to test for alcohols and other hydroxyl compounds.
Oxidation
Ethanol is oxidized by strong oxidizing agents such as warm acidified potassium dichromate or acidified potassium permanganate to ethanoic acid.

The Uses of Alcohol
Explain the uses of alcohol
Alcohol is used as an alcoholic beverage. Different alcohol drinks contain different amounts of ethanol. Beer and wines contain 8–13% of ethanol. Sprits such as whisky, gin, brandy, rum, etc. contain about 35–40% of ethanol. However, these beverages must only be drunk with moderation as too much consumption of alcohol can lead to heath problems.
Ethanol as fuel.
- Ethanol burns with a clear flame, giving out quite a lot of heat.C2H5OH(l) + 3O2(g) → 2CO2(g) + 3H2O(g) + heat On a small scale, ethanol can be used as methylated spirit (ethanol mixed with methanol or other compounds) in spirit lamps and stoves.
- Ethanol produced by fermentation of sugar from sugar cane has been used as an alternative fuel to gasoline (petrol), or mixed with gasoline to produce “gasohol”.
Ethanol as a solvent.
Alcohol is a good solvent, dissolving many compounds that are insoluble in water. The fact that it evaporates easily makes it a good solvent in products like glues, paints, varnishes, deodorants (perfumes), and aftershaves.
It is used in the manufacture of many chemicals
For example, it is used in making sweet–smelling liquids called esters which are used as solvents, in food flavourings, and as fragrance in beauty products (cosmetics).
Ethanol is also used as an ingredient in iodine tincture (a mixture of alcohol and iodine) which is largely used in hospitals for treatment of wounds.
The Harmful Effects of Alcohol
Explain the harmful effects of alcohols
In spite of its widest use as a useful product, ethanol, taken as an alcoholic beverage, has a lot of detrimental health effects. Some of the effects of alcohol are as follows:
- Even just one drink impairs coordination and judgement. It is a factor among the many causes of road accident as it leads to blurred vision.
- It leads to lack of muscular control (e.g. drunken stagger) and ultimately to coma, the state in which a person is said to be dead–drunk.
- Prolonged consumption of too much alcohol causes liver deterioration (cirrhosis of the liver) which can cause liver failure and death. Also heavy drinking eventually damages the muscle tissue of the heart. There may well be some long–term damage to the brain. All these health effects accelerate death.
- Alcohol is a depressive drug and can be addictive. Occasional drinking may lead to alcohol addiction, a condition during which a person is said to be alcoholic. When a drunkard finds him/herself in such a condition it is very difficult to go without drinking. This can eventually lead to poverty as a drunkard spends most of his/her time and money drinking (buzzing).
- Alcohol can make someone aggressive. This accounts for many arrests, conviction and jail sentences.
- Excessive drinking causes depression and other mental disorders.
- It can lead to gastric ulcers, high blood pressure and cancer of the mouth, throat, and gullet. People who smoke as well are at greater risk from thesecancers.
Women are at greater risk than men from the harmful effects of alcohol. One reason is that men‟s bodies have higher water content. So alcohol in their body fluids is more diluted.
Drinking during pregnancy can damage the baby‟s brain and heart, and slow down its growth so that it is born underweight.
Carboxylic Acids
Carboxylic acids form a homologous series of the general formula CnH2n+1COOH (or CnH2n+1CO2H), where n = 1, 2, 4, etc. for successive members of the group. All these acids have the characteristic functional (carboxyl) group, –COOH, attached to a hydrocarbon chain.
Natural Sources of Organic Acids
Identify natural sources of organic acids
There are various natural sources of organic acids. Some of these sources are:
- milk (lactic acid)
- citrus fruits (citric acid);
- tobacco (nicotinic acid); and
- tea (tartaric acid).
The Oxidation of Ethanol to Ethanoic Acid
Explain the oxidation of ethanol to ethanoic acid
When exposed to open air, ethanol is oxidized (by oxygen of the air) to ethanoic acid. The reaction for the process occurs thus:

The Structures of the Homologues of Carboxylic Acids up to Five Carbon Atoms
Write the structures of the homologues of carboxylic acids up to five carbon atoms
Carboxylic acids are named as if they are derived from alkanes by the replacement of one hydrogen atom by the –COOH group. The two lowest members, containing one atom and two carbon atoms respectively, are:
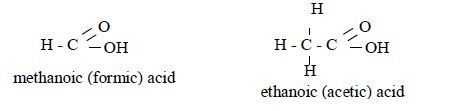
The other members of the homologous series are as shown below:
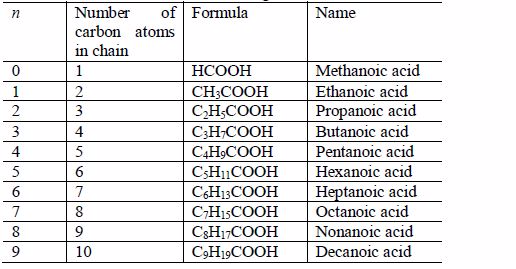
The successive members of the series have molecular formulae which differ by –CH2 It is important to remember that every carboxylic acid molecule contains the functional group –COOH which is called the carboxyl group.
The Isomers of Carboxylic Acids up to Five Carbon Atoms
Name the isomers of carboxylic acids up to five carbon atoms
Like other organic compounds, carboxylic acids also exhibit isomerism. Isomers of carboxylic acid are a result of branching of the hydrocarbon end (R) rather than the position of the carboxyl group in a molecule of the carboxylic acid. More isomers of the carboxylic acids can be created by branching the hydrocarbon end in as many different ways as possible.
Rules
The carbon of the carboxyl group (–COOH) is considered as carbon atom number 1.
Identify the positions of the alkyl group(s) attached to the (longest) acid chain. For example, in a molecule,
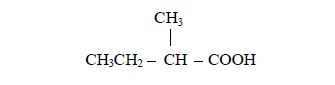
the alkyl group is methyl (–CH3) and it is attached to carbon number 2.
Name the branched alkyl group, followed by the name of the acid to which the alkyl group is attached. For example, in the case above (rule no.2):
- the alkyl group is methyl;
- it is attached to carbon number and
- the acid to which it is attached is butanoic acid,CH3CH2CH2COOH
Therefore, the name of the compound is 2-methylbutanoic acid.
In case there occurs more than one alkyl groups in the compound the prefixes di(2), tri(3), tetra(4) etc (as it was the case in alkanes) may be used. For, example in the compound
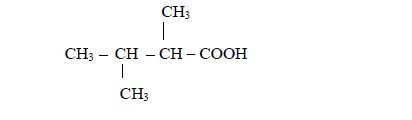
- there are two methyl groups, one attached to carbon number 2 and the other to carbon number 3; and
- they are both attached to butanoic acid chain.
Therefore, the name of the compound is 2,3-dimethylbutanoic acid.
Isomerism and nomenclature
Branching isomerism is found in this homologous series. Isomerism in carboxylic acids begins from butanoic acid, C3H7COOH. The first three members of the series do not show isomerism because their hydrocarbon ends do not form branches. The following are the structures and names of the isomers of carboxylic acids up to five carbon atoms:
- Butanoic acid, C3H7COOH or C3H7CO2H or CH3CH2CH2COOH
Isomers:
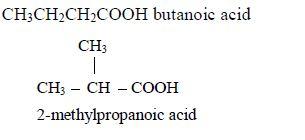
- Pentanoic acid, C4H9COOH
Isomers
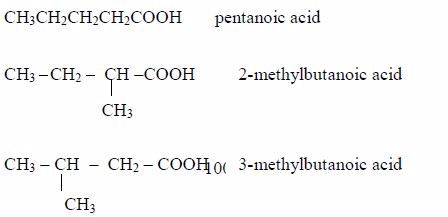
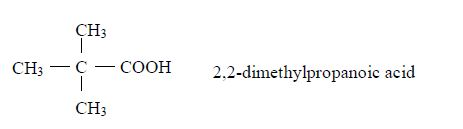
The Properties of Carboxylic Acids
Explain the properties of carboxylic acids
Carboxylic acids are weak acids. They are slightly ionized in dilute solutions.

Like inorganic acids, their solutions contain H+ ions. The presence of H+ ions give the solutions acidic behaviour, that is, their solutions affect indicators, just like the inorganic acids do.
Neutralization
Like inorganic acids, carboxylic acids react with metals, alkalis, carbonates, and hydrogen carbonates to form salts. For example:
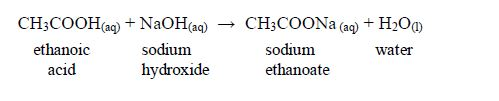
Esterification
The reaction between carboxylic acids and alcohols is called esterification. The acids will react reversibly with alcohols to form sweet–smelling esters. Concentrated sulphuric acid is a catalyst for the reaction.

The reaction can be reversed to recover an acid and alcohol again by boiling the products (an ester + water) with a mineral acid (HCl or H2SO4) or with an aqueous alkali (KOH or NaOH) as a catalyst.
Esters are manufactured for use as solvents, food flavourings, and fragrance for perfumes and beauty products. Ethyl ethanoate is just one example of many esters. The esters usually have strong and pleasant smells. Many of these compounds occur naturally. They are responsible for the flavours in fruits and for the scents of flowers. Fats and oils are naturally occurring esters used for energy storage in plants and animals. Some of the naturally occurring esters include:
- vegetable oils e.g. palm oil, groundnut oil, cashewnut oil, olive oil, sunflower oil, etc; and
- animal fats.
All esters contain the functional group,
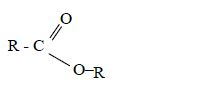
where R is any alkyl group.
Preparation of Soap from Animal Fats or Vegetable Oil
Prepare soap from animal fats or vegetable oil
Vegetable oils are formed from fatty acids and an alcohol called glycerol (also called glycerine). Fatty acids are carboxylic acids with long chains of carbon atoms. They are called “fatty” because the long chains repel water, making them immiscible with water. Glycerol or glycerine (or propane–1,2,3-triol) has three –OH groups. This is how fatty acids and glycerol react:
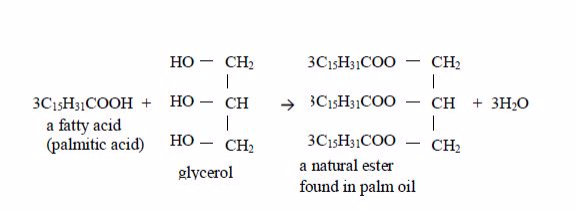
Preparation of soap from oils
Soap is made by heating animal fats or vegetable oils with sodium hydroxide solution. The oils react with the solution of sodium hydroxide and break down to form glycerol and the sodium salts of their fatty acids. These salts are used as soap. The reaction equation is:
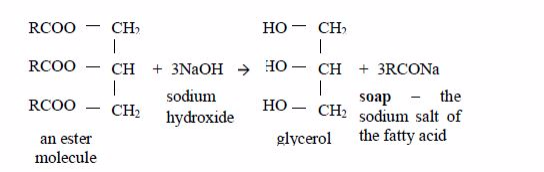
This process is known as saponification. The soap you buy is made from a blend of different oils. When soap dissolves in water it ionizes thus:

The cleansing agent in soap is the ion, RCOO–
No comments:
Post a Comment
Note: Only a member of this blog may post a comment.